CRISPR and the brain
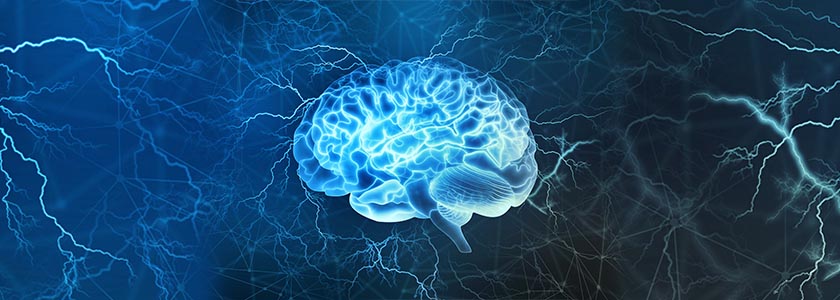
From erasing fearful memories in rats to engineering decaf coffee beans, feats of CRISPR gene editing can often sound like science fiction. But this method isn’t just about creating spicier tomatoes or greener fuel; CRISPR also holds potential applications in neuroscience—both as a research tool and, hopefully, a treatment for neurological diseases and disorders.
The CRISPR-associated protein (CRISPR-Cas) system offers the potential to greatly improve basic and translational neuroscience research. But, in terms of creating personalized brain therapy using these gene editing tools, are we there yet?
While the molecular mechanisms of several brain functions remain poorly understood, advances in next generation sequencing (NGS) and CRISPR applications have filled in some of these gaps. Recent research successes, such as the intracranial injection of CRISPR-Gold for a study on fragile X syndrome—a genetic condition causing mild to moderate intellectual disability—may contribute to the development of personalized, brain-targeted therapies.
Current challenges for CRISPR research and neuroscience
Despite the promising research trajectory, scientists have simultaneously discovered that the DNA-cutting machinery of the CRISPR system, the Cas enzyme, doesn't necessarily translate easily when applied to the brain and mental illnesses. This is partly because stem cells (and neurons generated from them for research) have a very active response to DNA damage; even a single DNA cut from Cas9 can lead to toxicity resulting in cell death. This points to how paramount the genetic engineering of complexes is which can access the central nervous system with the least potential harm, cellularly or otherwise.
Gene regulatory mechanisms are key for successful coordination and functionality of the nervous system. This carefully initiated regulation system ensures the essential structural, physiological, and functional properties of all neuron circuitry. The question of attempting brain-based therapy arises with any resulting dysregulation of these carefully integrated processes. One major aspect of such cellular regulatory mechanisms is epigenetics, or heritable phenotype changes that do not involve alterations in the DNA sequence. These can affect how cells read genes, and impact which ones are turned on or off.
Whether the issue stems from epigenetic patterns, or from a transcriptional dysregulation, the resulting damage can impact neural plasticity (the brain’s ability to change and adapt throughout a lifetime), memory functionality, and behavioral changes tied to experience. With this in mind, it is not hard to imagine how faulty gene expression becomes relevant to a variety of psychiatric and neurodegenerative conditions.
Using CRISPR activation (CRISPRa), researchers in March 2020 published in the journal Brain: A Journal of Neurology, that they were able to demonstrate the ability to upregulate an endogenous ion channel in vitro and in vivo in mice. In other words, CRISPRa was able to drastically change the firing rate of neurons, revealing important aspects of neural network behavior regarding seizure patterns related to epilepsy. Such a treatment option could be realistic as a safe alteration tool for neuron activity, without initiating harmful protein accumulation, toxicity, and cell death. Potentially, this type of gene expression regulation could be used to treat other neurological diseases with altered transcription.
CRISPR reagents and the blood-brain barrier
A major issue that arises when designing a personalized therapy for brain disorders is the ability to pass through the blood-brain barrier (BBB) successfully. The BBB functions to isolate and protect neural tissue and controls the entry of molecules from blood to brain. By default, these functions hinder molecule-based therapy attempts.
This is relevant to gene editing in that the blood-brain barrier (BBB) makes it hard to get CRISPR reagents into the brain. So far, current research attempts for BBB methods include in vitro (static and dynamic), in vivo (microdialysis), and in situ (brain perfusion). Nanoparticle delivery is an option currently being explored as well.
One well-documented therapy issue with the BBB is in the case of Parkinson’s disease. Patients with Parkinson’s are usually treated with the dopamine replacement agent levodopa (L-DOPA), which can alleviate some of the symptoms of neurodegeneration for a few years.
Unfortunately, the continuous treatment of synthetic dopamine capable of crossing the BBB results in changes to cellular transcriptional behavior over time. Because of this, and thanks to DNA methylation, the prolonged use of L-DOPA eventually causes dyskinesias (involuntary, uncontrolled muscle movements) and adversely impacts personality. These less-than-ideal outcomes are due to continuous changes being made to regulation, establishing the need for therapies that surpass the BBB problem without harming neural system integrity.
Identifying the genes causing brain disorders such as schizophrenia, autism, and depression
For many common neurodegenerative diseases, sporadic factors are the most common causality. A majority of patients with Parkinson's do not carry rare familial genetic mutations; instead, they tend to have sporadic, or non-familial expression of the disease. Nonetheless, they still feature excess misfolded alpha-synuclein protein and significant loss of dopaminergic neurons, leading to the same presentation of the disease as those with familial genetic ties. This unhappy reality has made the successful discovery of a biomarker to test for an individual’s risk a persistent challenge.
For a number of other brain disorders such as autism, schizophrenia, OCD, and depression, there are hints at the affected genes responsible. Besides trying to pinpoint the exact gene or genes, identifying the brain circuits affected by the mutated genes linked to each psychiatric issue is equally important. An example of how this type of challenge may be unraveled was on display when MIT neuroscientists discovered that two different mutations of the Shank3 gene had separate roles in autism and schizophrenia—two very different disorders.
CRISPR will likely become more useful for hereditary conditions stemming from a dominant gene, like with Huntington’s disease (HD), than for non-hereditary diseases. In theory, if the HD’s dominant gene is successfully knocked out or made inoperative, the healthy gene could be read for instructions instead.
With CRISPR technology, gene editing could be an amazing option for the treatment of neurodegenerative, mental illnesses, and possibly even psychiatric disorders. Perfecting this style of biological engineering could result in opportunities to eliminate anomalous genes—if only we can ensure the specificity and precision required for complex neural systems, eventually.